Next-Gen Battery Innovations: Transforming Electric Vehicle Technology
Written on
Chapter 1: Lithium Battery Innovations
Recent advancements indicate that ultra-fast batteries are approaching commercial viability. This innovative research tackles the issues of short-circuiting and failure commonly associated with traditional lithium metal batteries. Currently, lithium-ion batteries dominate the electric vehicle (EV) market. However, they face significant challenges, such as restricted driving range, performance deterioration, and safety issues, which hinder their broader acceptance. Fortunately, scientists are actively developing safer, high-performance alternatives.
Among the most promising developments is the solid-state lithium-ion battery. This technology has the potential to transform energy storage by offering a safer, more efficient, and environmentally friendly option for electric vehicles and other applications. Nevertheless, there are still several technical challenges to overcome before mass production can begin.
Description: This video discusses the breakthrough in solid-state batteries that could significantly enhance the capabilities of electric vehicles in 2024.
Visualizing Electric Vehicle Growth
The surge in EV adoption observed in 2021 suggests that this trend will continue to accelerate.
Despite the advantages of fast-charging lithium metal batteries with solid electrolytes, their performance has not met expectations. While these batteries are lightweight and non-flammable, they have encountered significant issues with short-circuiting, which hinders their widespread adoption. Researchers from Stanford University and SLAC National Accelerator Laboratory now claim to have addressed this problem.

According to William Chueh, a senior author of the study, "Even a slight indentation, bending, or twisting of the batteries can lead to microscopic fissures in the materials, allowing lithium to penetrate into the solid electrolyte and cause short-circuiting."

The primary issue stems from mechanical stress, especially during rapid recharging. This is why EV manufacturers advise against frequent use of superchargers. However, researchers emphasize that other factors, such as dust or impurities introduced during production, can also contribute to battery failure.
The challenge of solid electrolyte failure is not new and has been explored in numerous studies. Various theories have been proposed regarding its causes, including the unintended flow of electrons and chemical interactions. To investigate these phenomena, the researchers conducted extensive experiments, demonstrating how nanoscale defects and mechanical stress lead to solid electrolyte failures.
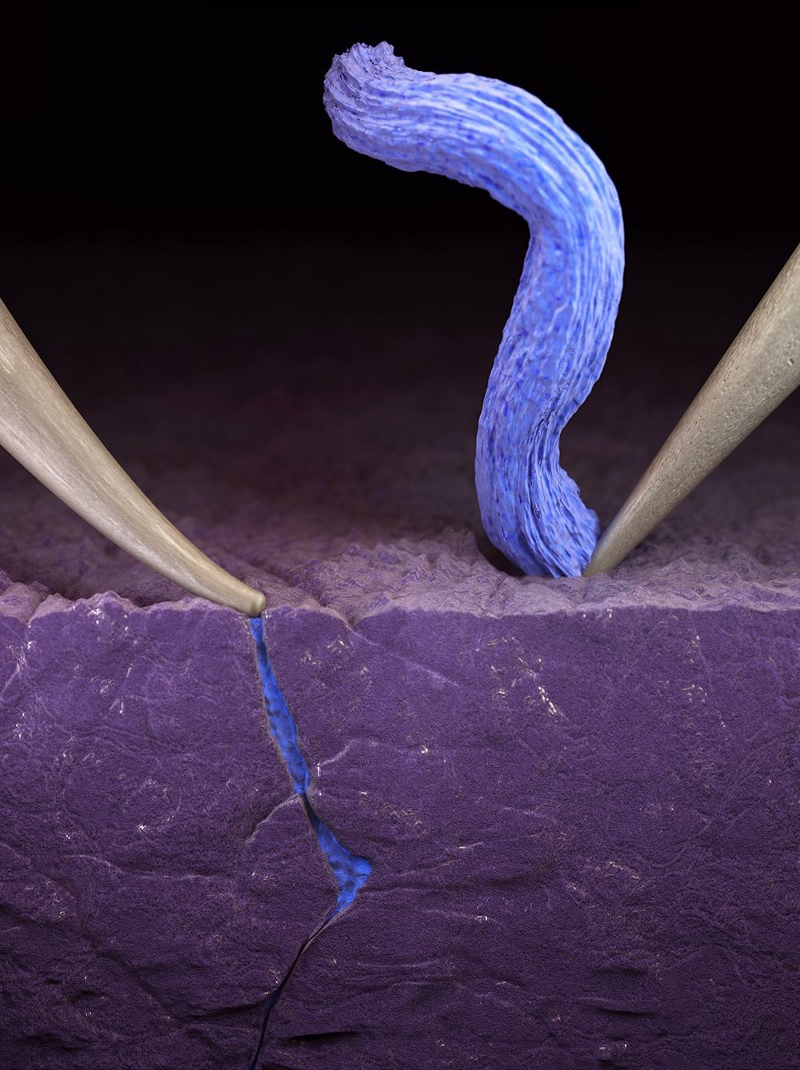
This illustration depicts how applied pressure can cause fractures in the solid electrolyte while lithium accumulates. The right side shows the desired outcome where the probe does not press against the electrolyte.
Global researchers working on innovative solid electrolyte rechargeable batteries can utilize the findings from this Stanford study to advance their own work. The development of energy-dense, fast-charging, and non-flammable lithium metal batteries could help overcome the main obstacles to the widespread adoption of electric vehicles.
Many current solid electrolytes are made from ceramics, which effectively separate the two energy-storing electrodes while allowing for swift lithium ion transport. Importantly, these materials are fire-resistant. However, like common ceramics, they can develop tiny surface cracks. The researchers conducted over 60 experiments, revealing that ceramics often exhibit microscopic fissures, some less than 20 nanometers wide—significantly smaller than a human hair.
Chapter 2: Mechanical Stress and Battery Performance
Description: This video covers three new futuristic battery technologies for 2024, including proof of concept from Elon Musk.
The research team found that these inherent cracks can expand during rapid charging, allowing lithium to infiltrate. By using an electrical probe to create a miniature battery, they monitored the rapid charging process with an electron microscope. They also employed an ion beam to analyze why lithium deposits on some areas of the ceramic electrolyte while penetrating others, leading to short circuits.
The primary factor affecting this process appears to be pressure. When the electrical probe lightly touches the electrolyte surface, lithium accumulates evenly, even during rapid charging. However, when pressure simulating mechanical stress is applied, the risk of short-circuiting increases.
A practical solid-state battery consists of several layers of cathode, electrolyte, and anode sheets stacked on top of each other. The electrolyte serves as a barrier, allowing lithium ions to move freely between the cathode and anode. If the two electrodes make contact due to a metallic lithium bridge, a short circuit can occur.
With their new insights, Chueh’s team is exploring ways to intentionally apply mechanical forces to enhance the durability of the material during production, akin to the methods used by blacksmiths to strengthen blades. They are also investigating techniques to coat the electrolyte surface to prevent or repair cracks as they form.
The complete findings of this research have been published in the Journal of Nature Energy.